Periodic Table |
![]() |
![]() |
![]() |
![]() |
![]() |
![]() |
![]() |
Nucleosynthesis of The Elements
This page is concerned with where the chemical elements come from: how atomic nuclei are forged. It is a long, complicated story, largely deduced in the second half of the twentieth century, that romantically concludes: We Are Stardust.
The Start: Big Bang Nucleosynthesis
Current thinking is that the the universe erupted from the cauldron of the Big Bang some 13.8 billion years ago, as described on this Wikipedia timeline page.

And from NASA:
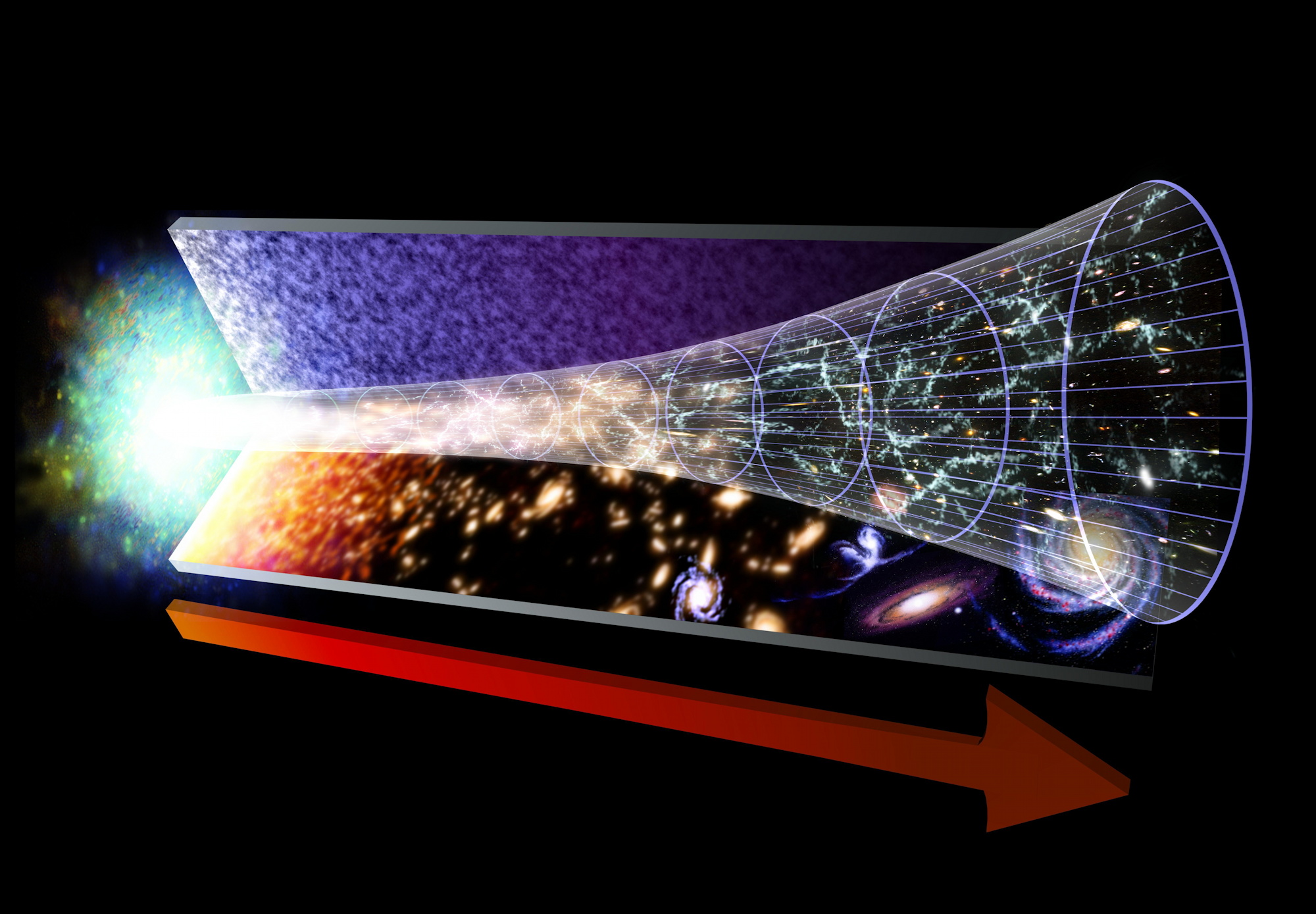
Big Bang Nucleosynthesis
The period of baryionic matter formation: protons, neutrons and some of the lighter elements – the epoch of Big Bang Nucleosynthesis (BBN) – lasted from 10 seconds to ~20 minutes from the beginning itself.
During this period:
- The quark-gluon plasma (quark soup) cooled to an ionised plasma
of: photons, electrons, positrons, neutrinos, protons and neutrons. Initially the temperature
was so high that the protons and electrons could combine into neutrons:
proton + electron ⇄ neutron
- Equilibrium meant that both protons and neutrons were present
in large numbers.
- The universe expanded
and cooled, to ~1010 Kelvin. At this temperature the nuclear chemistry
changed and no more neutrons were formed. Free neutrons have a half
life of 617 seconds. Once they stopped being produced [by the above equilibrium] their numbers,
relative to the stable protons, started to decline.
- When the universe had cooled to ~109 Kelvin, there were 164 neutrons to every 1000 protons. At this lower temperature
neutrons were able to combine/react with protons to form deuterium nuclei, 2H. In this bound state neutrons are stable and no longer decay.
- In nuclear chemistry
terms, deuterium nuclei, 2H, are very reactive. For several minutes the deuterium nuclei, 2H, reacted by a variety of nuclear reactions to give a mixture
of isotopes: 3He, 4He, 7Li, along with
the primordial 1H and 2H:
A graph, from astro.ucla.edu, shows the (log) time evolution of the abundances of the light elements:

Two linked 'how science works' points:
The ratios of 1H, 2H, 3He, 4He and 7Li in the early universe can be measured by astronomers – with considerable difficulty – and the numbers obtained constrain the mass, temperature and density conditions at this epoch.
The nuclear chemistry described above is confirmed by high energy physics experiments at CERN, the Stanford Linear Accelerator and a few similar establishments that can reproduce the conditions fractions of a second after the Big Bang, albeit on a small scale. This science is all part of the standard model of contemporary physics.
As far as chemists are concerned, little else happened for several hundred thousand years after this crucial epoch.
- The universe expand outwards and cooled and nuclear chemistry ceased.
- After about 300,000 years the process of recombination occurred.
- The expanding universe had been an optically opaque plasma of photons,
free electrons and 1H, 2H, 3He, 4He & 7Li nuclei. But when the temperature fell to about 3000°, the electrons were able to combine with the atomic nuclei to form neutral atoms, and the universe became optically clear.
Stellar Nucleosynthesis
This would be the end of the story, except that the rapidly expanding universe had a built in brake – gravity, the great sculptor – which operated both globally and locally. The implications of gravity for the entire universe are still the subject of debate, but local effects are better understood. After about 100 million years gravity caused and still causes matter to collapse into bodies that become hot and light up the dark sky as stars.
Stars are hot and dense enough to burn hydrogen, 1H, to helium-4, 4He, and heavier nuclei.
There are several nuclear synthetic routes: – CNO cycle, Triple α & pp-chain:
As a result a variety of atomic nuclei are formed, including:
8Be, 13N, 13C, 14N, 15O, 15N, 12C, 16O & 17F
although many of these nuclei are either radioactive or are quickly consumed in the stellar furnace.
Stars evolve so that they have onion-skin like shells of thermonuclear combustion with differing nuclear chemistry. The exact structure depends on the mass of the star, from here:
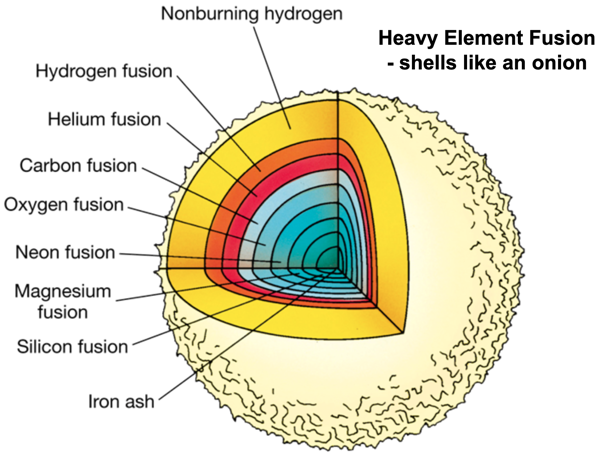
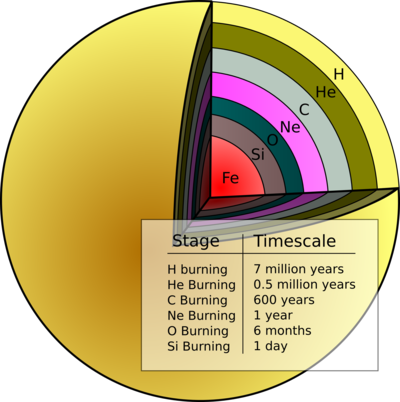
The temperature in the stellar interior increases and more nuclear synthetic pathways become available producing:
20Ne, 23Na, 23Mg, 24Mg, 28Si, 31P, 31S, 32S... and all the way up to 56Fe
Supernovae
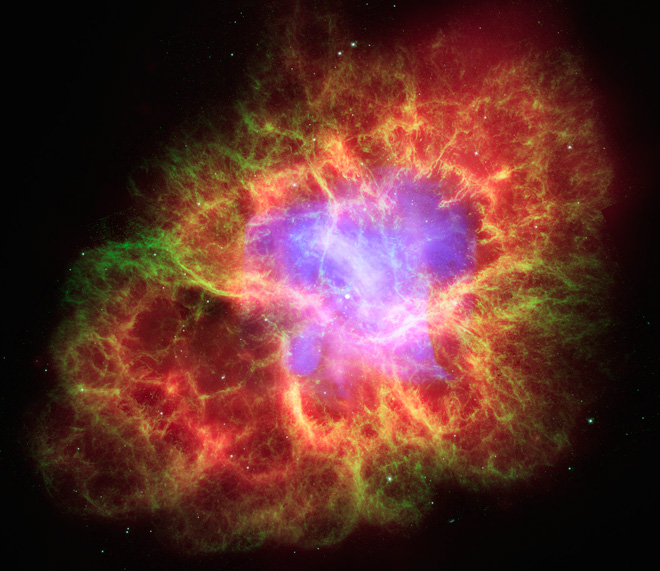
The chemical elements are ejected into space by several processes, each involving a dying star:
- Main
sequence stars like our Sun burn out and become cold white dwarves.
But before they die, they go through a red giant stage where the outer
mantle layers, enriched in elements like oxygen, nitrogen and carbon often in the
form of nanometre size diamonds are quietly 'blown off' into the
interstellar medium.
- Stars eight times heavier than our Sun explode as a super nova. Due to the thermochemistry of the various nuclear processes, each shell of nucleosynthesis proceeds on an accelerating time scale and Si burns to Fe in hours. Conditions in the core become so extreme that electron pressure is overcome and the protons are forced to react with electrons to give neutrons
proton + electron → neutron + neutrino
and a neutron star is born in less than a second. The rebounding shock wave plus radiation pressure from the escaping neutrinos causes the outer layers star to explode outwards as a Type II supernova.
These conditions have a massive flux of free neutrons and the various nuclei are able absorb one or more of these neutrons, undergo beta decay, absorb another neutron or neutrons, another beta decay... a process which moves nuclei up (heavier) the periodic table towards and past uranium.
- The
third process is the Type Ia supernova. This occurs when a white dwarf
is held in a tight binary association with a main sequence star. The
small, dense white dwarf pulls the surface layers from the companion
star until enough mass builds so that a runaway thermonuclear incineration
occurs on the surface of the white dwarf which explosively disassembles...
- During a supernova, the process of neutron capture builds up elements as far as Z=100, Ferminium. The nucleus 257Fm has a half-life of a few months, but apparently the process of nucleosynthesis can go no further. [Some Nucleosynthesis Effects Associated with r-Process Jets, Astrophysical Journal 2003, 587: 327-340]. Thanks to the Marks Bros for the tip... their periodic table formulation uses this information.
- It is now thought [writing in 2017] that merging neutron stars are a major source of heavy r-process elements. Among several options, such mergers can/may produce a black hole, but there will also be a great deal of material ejected, and this material will be subject to a high neutron flux.
This appears to have been confirmed in the 100 second LIGO observation of mid-2017, which was also observed by optical telescopes.
Whatever the mechanism, shells of debris consisting of a isotopic zoo of atomic nuclei are ejected into the interstellar medium. Over millions of years this material cools to a mildly radioactive clinker that collects together by gravity... where it participates in next generation of star formation.
For reasons not yet fully understood, the contracting cloud of hydrogen, helium and metals astronomers regard all elements other then H and He as metals evolves to form a disk of planets around a central star.
The inner planets of our solar system are not massive enough to have sufficient gravity to hold onto geseous hydrogen, H2, and helium, and these gases escape into space leaving the Earth depleted in these elements, but enriched in heavy elements with respect to the universe as a whole. Our planet is large enough so that the residual radioactivity, mainly from 40K, is able to heat the mantle so that it remains hot and fluid.
Some hydrogen remains on Earth by chemically combining with oxygen and trapped as water, a substance essential for our planet's biology. When the Earth formed much of the hydrogen was combined with carbon as methane, CH4, however this inorganic methane a comparatively rare substance today.
Hard Data
- The universe 13.8 billion years old
- After about 300 million years the galaxies appeared. (This is an area of active research due to recent findings from the JWST).
Composition of the universe:
- 73% dark energy
- 23% dark matter
- 4% matter: stars, planets, interstellar dust
- 200 billion galaxies in total in the observable universe
- 200 billion stars per galaxy
- During the lifetime of our local galaxy there have been about 100 million supernova explosions. Their frequency and yield are consistent with the observed abundance of elements.
- Most of the iron on the Earth was produced in Type Ia supernovae events, most of the oxygen in Type II supernovae explosions and most of the gold in neutron star mergers: We Are Star Dust
Graphical Timeline

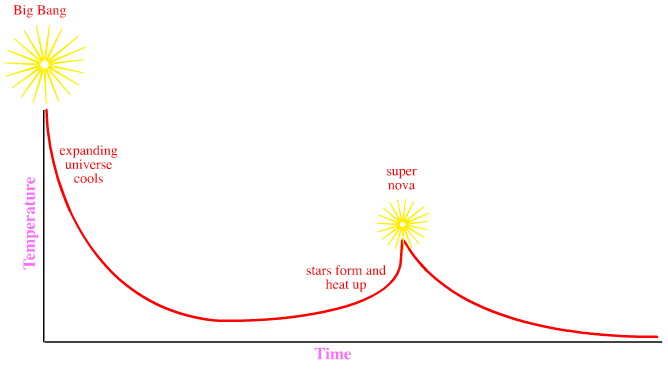
Some Amazing Scale Images
When we look at the night sky we see dots of light, but these come from a heterogeneous group of stellar entities. All stars more than eight times more massive than the sun are destined to explode, the big ones all go bang!
The nucleosynthesis reactions discussed on this page can be found in The Chemical Thesaurus.
The main reference for this page: Intro to Modern Astrophysics by Carroll & Ostlie, Addison-Wesley (1996) as well as the Wikipedia.
![]() |
![]() |
![]() |
Introduction to Chemogenesis | Segrè Chart |
© Mark R. Leach 1999-
Queries, Suggestions, Bugs, Errors, Typos...
If you have any:
Queries
Comments
Suggestions
Suggestions for links
Bug, typo or grammatical error reports about this page,please contact Mark R. Leach, the author, using mark@meta-synthesis.com
This free, open access web book is an ongoing project and your input is appreciated.