Periodic Table |
![]() |
![]() |
![]() |
![]() |
![]() |
![]() |
![]() |
The INTERNET Database of Periodic Tables
There are thousands of periodic tables in web space, but this is the only comprehensive database of periodic tables & periodic system formulations. If you know of an interesting periodic table that is missing, please contact the database curator: Mark R. Leach Ph.D.
Use the drop menus below to search & select from the more than 1300 Period Tables in the database:
- SEARCH:
- By Decade
- By Type
-
Pre-Selected
Best Four Periodic Tables for Data All Periodic Tables by Name All Periodic Tables by Date All Periodic Tables by Reverse Date All Periodic Tables, as Added to the Database All Periodic Tables, reverse as Added Elements by Name Elements by Date Discovered Search for: Mendeleev/Mendeléeff Search for: Janet/Left-Step Search for: Eric Scerri Search for: Mark Leach Search for: René Vernon Search for: Electronegativity
-
By Year
2020 2019 2018 2017 2016 2015 2014 2013 2012 2011 2010 2009 2008 2007 2006 2005 2004 2003 2002 2001 2000 1999 1998 1997 1996 1995 1994 1993 1992 1991 1990 1989 1988 1987 1986 1985 1984 1983 1982 1981 1980 1979 1978 1977 1976 1975 1974 1973 1972 1971 1970 1969 1968 1967 1966 1965 1964 1963 1962 1961 1960 1959 1958 1957 1956 1955 1954 1953 1952 1951 1950 1949 1948 1947 1946 1945 1944 1943 1942 1941 1940 1939 1938 1937 1936 1935 1934 1933 1932 1931 1930 1929 1928 1927 1926 1925 1924 1923 1922 1921 1920 1919 1918 1917 1916 1915 1914 1913 1912 1911 1910 1909 1908 1907 1906 1905 1904 1903 1902 1901 1900 1899 1898 1897 1896 1895 1894 1893 1892 1891 1890 1889 1888 1887 1886 1885 1884 1883 1882 1881 1880 1879 1878 1877 1876 1875 1874 1873 1872 1871 1870 1869 1868 1867 1866 1865 1864 1863 1862 1861 1860 1859 1858 1857 1856 1855 1854 1853 1852 1851 1850 1844 1843 1842 1838 1836 1831 1830 1829 1825 1824 1817 1814 1813 1811 1808 1807 1804 1803 1802 1801 1800 1798 1794 1791 1789 1787 1783 1782 1781 1778 1775 1774 1772 1771 1766 1753 1751 1748 1735 1718 1700 1690 1687 1682 1671 1669 1624 1617 1520 1000 -300 -450 -800 -1000 -2000 -3500 -3750 -5000 -6000 -7000 -9000
Periodic Table database entries referencing the term electronegativity, by date:
Year: 1836 | PT id = 453 |
Berzelius' Electronegativity Table
Berzelius' electronegativity table of 1836.
The most electronegative element (oxygen or Sauerstoff) is listed at the top left and the least electronegative (potassium or Kalium) lower right. The line between hydrogen (Wasserstoff) and gold seperates the predomently electronegative elements from the electropositive elements. Page 17 and ref. 32 from Bill Jensen's Electronegativity from Avogadro to Pauling Part I: Origins of the Electronegativity Concept, J. Chem. Educ., 73, 11-20 (1996):
Year: 1870 | PT id = 454 |
Baker's Electronegativity Table
Baker's electronegativity table of 1870 differs from Berzelius' listing of 1836 only by the addition of the newly discovered elements. Page 280 and ref. 5 from Bill Jensen's: Electronegativity from Avogadro to Pauling Part II: Late Nineteenth- and Early Twentieth-Century Developments, J. Chem. Educ., 80, 279-287 (2003):

Year: 1886 | PT id = 798 |
Discovery of Fluorine
F
Fluorine, atomic number 9, has a mass of 18.998 au.
Fluorine exists as a pale yellow diatomic molecular gas, F2. It is the most electronegative and reactive of all elements: it which reacts with practically all organic and inorganic substances.
Fluorine was first observed or predicted in 1810 by A.-M. Ampére and first isolated in 1886 by H. Moissan.
Year: 1888 | PT id = 1267 |
Stoney's Spiral Periodic Table
In the Proceedings of the Royal Society of London, Series A, Containing Papers of a Mathematical and Physical Character, Volume 85, Issue 580, Aug 1911, p. 472, there is an article On Dr. Johnstone Stoney's Logarithmic Law of Atomic Weights, by Lord Rayleigh (who co-discovered argon in 1894), who writes :
"In the year 1888, Dr. G. Johnstone Stoney communicated to the Society a memoir with title nearly as above, which, however, was not published in full. At the request of the author, who attaches great importance to the memoir, I have recently, by permission of the Council, consulted the original manuscript in the archives of the Society, and I propose to give some extracts, accompanied by a few remarks. The author commenced by plotting the atomic weights of the elements taken as ordinates against a series of natural numbers as abscissæ. But a curve traced through the points thus determined was found to be 'one which has not been studied by mathematicians.
"This sudden transition may have some connection with the fact that no elements have been found on sesqui-radius 16, although the investigation in § 3 shows that the values of m corresponding to the stations on sesqui-radius 16 cannot be dispensed with.
"The vacant places here pointed out are now occupied by the since discovered inert gases. The anticipation is certainly a remarkable one, and it goes far to justify the high claims made for the diagram, as representing in a telling form many of the leading facts of chemistry."
Comment from Mark Leach:
"Notice how the electronegative elements are positioned top right & bottom right and the electropositive elements top left & bottom right."

René Vernon writes:
"Stoney has another article in the September 1902 edition of the The London, Edinburgh and Dublin Philosophical Magazine and Journal of Science, called Law of Atomic Weights, pp. 411–415. At the back of the journal is an updated fold-out version of Stoney’s table, image attached.
- Ar, Kr and Xe fit on the spiral, and on spoke 16.
- Neon fits on the spiral but is instead on spoke 8.
- Helium is on spoke 18 but is not on the spiral.
- The circle in the middle represents H (p. 414).
"On the page after the updated spiral, there looks to be some printed content, but it is hidden by what looks to be a folded over page."
Thanks to René for the tip!
Year: 1893 | PT id = 63 |
Rang's Periodic Arrangement of The Elements
P.J.F. Rang's The Periodic Arrangement of the Elements, Chemical News, vol. 67, p. 178 (1893)
Observing that that Rang's table has four 'groups': A, B, C & D, René Vernon writes:
- Group A contains the strongest positive elements; group D the strongest negative elements. At such an early date, it's odd to see groups 1 to 3 categorised together.
- Group B are the elements with high melting points; "they are all remarkable for their molecular combinations" (presuamably, a reference to multiple oxidation states). At one side of group B are the "anhydro-combinations", probably referring to the simple chemistry of Ti, Zr, [Hf] Nb and Ta being dominated by insoluble oxides. At the other side are the "amin, carbonyl, and cyanogen combination", probably a reference to the group VIII carbonyls, as metal carbonyls had only just been discovered. Ni is shown after Fe, rather than Co.
- Group C includes the "heavy metals that have low melting points"; an early reference to frontier or post-transition metals, as a category.
- Rang says: ...if groups A and D be split up vertically in respectively three and two parts, the table presents seven vertical groups, and horizontally seven more or less complete series. Each group in each of the series 2 and 3 are represent by one element... The octave appears both horizontally and vertically in the table.
- Rang's reference to Di as representing all the triads between Ba and Ta kind of works since Hf would go under Zr, and that would leave 15 Ln or five sets of three. Thus, something like this:
Gd occupies the central position among the Ln. This arrangement won't fit however unless Rang envisaged all 15 Ln occupying the position under Y. - The location of H over | Ga | In | Tl, appears strange... but the electronegativity of H (2.2) is closer to B (2.04) than it is to C (2.55).
From Quam & Quam's 1934 review paper.pdf
Year: 1895 | PT id = 368 |
Thomsen's Systematic Arrangement of the Chemical Elements
In 1895 the Danish thermochemist Hans Peter Jørgen Julius Thomsen proposed (Thomsen, J., 1895. Z. Anorg. Chem. 9, 190 & Chemical News, 72, 89–91, p. 90) a pyramidal/ladder representation.
Notice how this formulation identifies the electropositive & electronegative elements with respect to the periodic table, thirty years before Linus Pauling.
Year: 1923 | PT id = 1256 |
Deming's Periodic Table With Commentry by Vernon
René Vernon writes:
Deming's 1923 periodic table is credited with popularizing the 18-column form.
I now see Deming used different thickness sloping lines to represent the different degrees of similarity between the main groups and their corresponding transition metal groups.
- The line between Li-Na and group 11 is dashed, denoting the weakest relationship.
- Be-Mg are in group 2 The line between Be-Mg and group 12 is not dashed, denoting a stronger relationship.
- B-Al are in group 3
- The line between B-Al and Ga-In-Tl is thicker yet.
When I plot up to 20 chemical properties v Z going down these options I get the following values for the average smoothness of the trendlines:
- 73.5% for Li-Na-Cu(+2)-Ag(+1)-Au(+3) versus 84% for Li-Na-K-Rb-Cs
- 70% Be-Mg over Zn versus 85% for Be-Mg-Ca-Sr-Ba
- 81% for B-Al-Ga-In-Tl versus 88% B-Al-Sc-Y-La
I would have thought the smoothness for the line between Li-Na and Cu would be < 70%, consistent with Deming’s dashed line. But the thickness of the line would depend on what Deming took into account when he drew it. The common wisdom about groups 1 and 11 is that their similarities are: "confined almost entirely to the stoichiometries (as distinct from the chemical properties) of the compounds in the +1 oxidation state." (Greenwood & Earnshaw 2002, p. 1177). Kneen et al. (1972, p. 521) say that, "the differences between the properties of the group IA and IB elements are those between a strongly and weakly electropositive metal." On this basis I follow Deming’s dashed line. I’ve appended some notes about Group 1 and Group 11.
- Main group 4 is C-Si-Ge-Sn-Pb
- The line between Si and Ti-Zr-Hf is thick
- The line between N-P and V is less thick
- The line between O-S and Cr is less thick again
- The line between F-Cl and Mn is dashed
I have [calculated] a smoothness for C-Si-Ti-Zr-Hf of 86% versus 70% for C-Si-Ge-Sn-Pb. Since Ti shows some transition metal chemistry but not C-Si, it is perhaps plausible to keep C-Si-Ge-Sn-Pb together (as Deming did ).
Deming was a smart author. Nigh on a century later and the metrics check out.

More about group 1 and group 11
There may be a little more to the relationship between Li-Na & Cu-Ag-Au, than is ordinarily appreciated. For example:
- The resulting composite "group" has two electropositive metals and three more electronegative metals so its overall nature is more nuanced then purely group 1 or purely group 11
- The ionic radii of Li+ and Cu+ are 0.76 and 0.77 Å, and there is at least some discussion in the literature about substitution phenomena (Vasilev et al. 2019, p. 2-15; Udaya et al. 2020, p. 98; Kubenova 2021 et al.)
- Group 1 and 11 metal atoms form clusters relatively easily including Au_42+, Ag_64+, Rb_75+, Na_43+ (Mile et al. 1991, p. 134; Wulfsberg 2000, p. 631).
- In an organometallic context, Schade & Scheyler (1988, p. 196) wrote that, "There is much evidence that differences between group 1 and group 11 metals are not of principal but rather gradual manner."
- Although most nonmagnetic metals exhibit superconductivity it is significant that the Group 1 and 11 metals do not become superconducting at very low temperatures (Rao & Gopalakrishnan 1997, p. 398).
- Gold forms intermetallic compounds with all alkali metals (Schwerdtfeger et al. 1989. p. 1769)
References
- Greenwood NN & Earnshaw A 2002, Chemistry of the Elements, 2nd ed., Butterworth Heinemann, Oxford
- Kubenova et al. 2021, "Some thermoelectric phenomena in copper chalcogenides replaced by lithium and sodium alkaline metals", Nanomaterials 2021, vol. 11, no. 9. article 2238, https://doi.org/10.3390/nano11092238
- Mile et al. 1991, "Matrix-isolation studies of the structures and reactions of small metal particles", Farady Discussions, vol. 92, pp. 129–145 (134), https://doi.org/10.1039/FD9919200129
- Rao CNR & Gopalakrishnan J 1997, New Directions on Solid State Chemistry, 2nd ed., Cambridge University Press, Cambridge
- Schade C & Schleyer PVR 1988, "Sodium, potassium, rubidium, and cesium: X-Ray structural analysis of their organic compounds", Advances in Organometallic Chemistry, vol. 27, Stone FGA & West R (eds), Academic Press, San Diego, pp. 169–278
- Schwerdtfeger et al. 1989, "Relativistic effects in gold chemistry. I. Diatomic gold compounds.", The Journal of Chemical Physics, vol. 91, no. 3, pp. 1762–1774. https://doi.org/10.1063/1.457082
- Udaya et al. 2020, Metal sulphides for lithium-ion batteries, in Inamuddin, Ahmer & Asiri (eds), Lithium-ion batteries: Materials and applications, Materials Research Forum, Millersville PA, pp. 91–122
- Vasiliev AN et al. 2019, Low-dimensional Magnetism, CRC Press, Boca Raton
- Wulfsberg 2000, Inorganic chemistry, University Science Books, Sausalito, CA
Year: 1960 | PT id = 444 |
Pauling's Complete Electronegativity Scale
From The Nature of The Chemical Bond, 3rd Ed, pp 93, Pauling gives a periodic table showing the electronegativity of the elements.
Notice how the d block appears between groups 3 and 4 (13 & 14), rather than between groups 2 and 3 (2 & 13):
Year: 1971 | PT id = 205 |
Satz Reciprocal System Periodic Table
Developed in 1971 for my book The Unmysterious Universe, this periodic table is based on Dewey B. Larson's Reciprocal System of theory. The numbers below the symbols indicate the rotational displacement (spin numbers) of the atoms. The Roman numerals indicated divisions; the rows, 1B to 4B, are referred to as "groups" rather than as "periods." Note that we have the same trouble positioning hydrogen as does everyone else; here, I've put it over both the alkali metals and the halogens, because it acts both as electropositive (e.g., with respect to water) and electronegative (with respect to carbon).
Click here for larger PDF file.
Ronald W. Satz, Ph.D.
Transpower Corporation
www.transpowercorp.com
transpower.wordpress.com
transpower@aol.com
Year: 1987 | PT id = 743 |
Elsevier's Periodic Table of the Elements
Prepared by P. Lof is Elsevier's Periodic Table of the Elements.
This educational wall chart features the periodic table of the elements supported by a wealth of chemical, physical, thermodynamical, geochemical and radiochemical data laid down in numerous colourful graphs, plots, figures and tables. The most important chemical and physical properties of the elements can be found - without turning a page.
All properties are presented in the form of tables or graphs. More than 40 properties are given, ranging from melting point and heat capacity to atomic radius, nuclear spin, electrical resistivity and abundance in the solar system. Sixteen of the most important properties are colour coded, so that they may be followed through the periodic system at a glance. Twelve properties have been selected to illustrate periodicity, while separate plots illustrate the relation between properties. In addition, there are special sections dealing with units, fundamental constants and particles, radioisotopes, the Aufbau principle, etc. All data on the chart are fully referenced, and S.I. units are used throughout.
Designed specifically for university and college undergraduates and high school students, "Elsevier's Periodic Table of the Elements" will also be of practical value to professionals in the fields of fundamental and applied physical sciences and technology. The wall chart is ideally suited for self-study and may be used as a complementary reference for textbook study and exam preparation.
- atomic number
- standard atomic weight
- ground-state electronic configuration
- element symbol
- element name
- discoverer and year of discovery
- melting point; boiling point
- critical temperature
- molar enthalpy of atomization
- molar enthalpy of fusion
- molar enthalpy of vaporization
- atomic energy levels of the outermost three orbitals
- formal oxidation states
- selection of standard reduction potentials
- first, second & third molar ionization energies
- Pauling electronegativity
- Allred-Rochow electronegativity
- molar electron affinity
- molar volume
- crystal structures
- polymorphic transition temperatures
- atomic radius
- effective ionic radii
- volumic mass (density)
- electrical resistivity
- thermal conductivity
- abundance in the solar system
- abundance in the Orgueil meteorite
- abundance in the solar photosphere
- abundance in the continental crust
- abundance in the primitive mantle
- abundance in the oceanic crust
- naturally occurring isotopes
- mass number and representative isotopic composition
- molar heat capacity
- Debye temperature
- coefficient of linear thermal expansion
- price; annual mining production
- world reserve base
- nuclear spin and NMR receptivity
- Mossbauer active nuclides
- physical (standard) state
- metallic character
- abundance in food (human daily intake)
- principal hazardous property
- Other information: Aufbau principle, quantum numbers, orbitals and sequence of orbital filling; trivial group names; drawings of crystal lattice structures; 12 plots of a chemical/physical property against atomic number; 9 plots of a property against another property; list of SI units and SI prefixes; list of other units and their conversion to SI; list of fundamental physical constants; scheme of fundamental particles; list of radioisotopes with half-life longer than 5 days, presenting half-life and mode(s) of decay, indicating cosmogenic isotopes and isotopes produced by U-235 fission, as well as radioisotopes used in geochronology, pharmacology and nuclear medicine.
Thanks to Eric Scerri for the tip!
See the website EricScerri.com and Eric's Twitter Feed
Year: 1993 | PT id = 114 |
WebElements: The Periodic Table on The Web
Mark Winter's WebElements was started in 1993 when it was one of the first websites on the internet.
Mark Leach's Chemogenesis web book uses the WebElements periodic table as its master data source, and it does not attempt to duplicate it.
Abundance of
elements (Earth's crust) |
Electronegativities
|
Oxidation states
in compounds |
Year: 2003 | PT id = 311 |
Electronegativity Periodic Table
"This image distorts the conventional periodic table of the elements so that the greater the electronegativity of an atom, the higher its position in the table", here:
Year: 2003 | PT id = 123 |
Electronegativity Periodic Table
A periodic table showing electronegativity, "The ability of an atom to attract electron density from a covalent bond" (Linus Pauling). Blue elements are electronegative, red elements are electropositive, and purple elements are intermediate. Notice how hydrogen is intermediate in electronegativity between carbon and boron and is positioned above and between these elements:
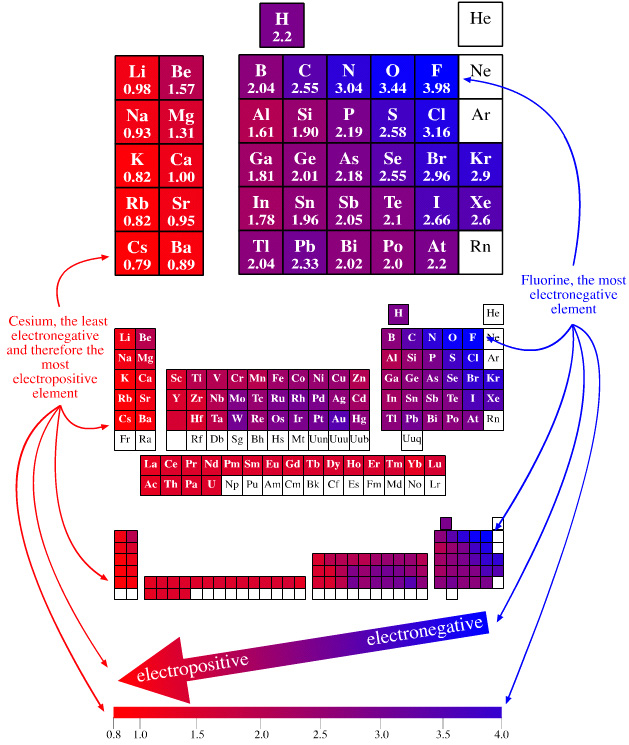
By Mark Leach
Year: 2005 | PT id = 137 |
Extraction from Ore to Pure Element
A periodic table showing how pure elements are extracted:
Highly electropositive elements (Na, K) and electronegative elements (Cl2, F2) can only be obtained by electrolysis.
By Mark Leach
Year: 2006 | PT id = 17 |
Where Should Hydrogen Go?
There are four possible positions for hydrogen:
- A Group 1 element, above Li, because it forms H+ ions.
- A Group 17 element, above F, because it forms H- ions.
- Above and between boron and carbon because it is of intermediate electronegativity.
- In the top middle, because nowhere else is ideal.
By Mark Leach
Year: 2006 | PT id = 56 |
Reaction Chemists' Periodic Table
OK, so which Is The Best formulation of The Periodic Table?
Personally as a reaction chemist, my preferred periodic table is the 'long' form shown below, with hydrogen above and between boron and carbon, although clearly other scientists have other ideas.
All periodic tables show the increase in mass and atomic number, Z, but only the long form unambiguously shows the general top-right-to-bottom-left trends in electronegativity, atomic radius, metallic properties and first ionisation energy.
Electronegativity is absolutely crucial to the understanding of structure, bonding, material type (van Arkel-Ketelaar triangle and Laing tetrahedron) and chemical reactivity, and it underpins much of the chemogenesis analysis.
Year: 2013 | PT id = 566 |
Electronegativity Chart (Leach)
From Mark R Leach's paper, Concerning electronegativity as a basic elemental property and why the periodic table is usually represented in its medium form, Journal & PDF.
Due to the importance of Pauling's electronegativity scale, as published in The Nature of The Chemical Bond (1960), where electronegativity ranges from Cs 0.7 to F 4.0, all the other electronegativity scales are routinely normalised with respect to Pauling's range.
When the Pauling, Revised Pauling, Mulliken, Sanderson and Allred-Rochow electronegativity scales are plotted together against atomic number, Z, the similarity of the data can be observed. The solid line shows the averaged data:
Year: 2013 | PT id = 610 |
Top 10 Periodic Tables
There are more than 1000 periodic tables hosted by the Chemogenesis Webbook Periodic Table database, so it can be a little difficult to find the exceptional ones.
Here we present – in our humble opinion – The ten most significant periodic tables in the database.
We present the best:
- Three best data rich periodic tables
- Five formulations which show the development of the modern PT
- One, of many, interesting alternative formulations
- One example of the periodic table being used as an infographic template
Three Excellent, Data Rich Periodic Tables
The first three of our top 10 periodic tables are classic element data repositories.
They all work in the same way: click on the element symbol to get data/information about the selected element. The three are Mark Winter's WebElements, Theo Gray's Photographic Periodic Table & Michael Dayah's Ptable.
- Since 1993 – and with its rather bland interface – WebElements has given access to vast quantities of in depth chemical data & information. This is the professional chemist's periodic table:
- Theo Gray's Photographic Periodic Table is undoubtedly the most attractive PT available in web space, but there is more. Clicking around the website gives access to a host of information, pictures & anecdotes from Theo's extraordinary and extensive collection of chemical elements:
- Ptable has a super-slick, and very fast interface. It is data/information rich and is available in 50 languages:
Five Formulations Showing The History & Development
The next five examples deal with history and development Periodic Table. The first is Dalton's 1808 list of elements, next is Mendeleev's 1869 Tabelle I, then Werner's remarkably modern looking 1905 formulation. This is followed by Janet's Left Step formulation and then a discussion of how and why the commonly used medium form PT formulation, is constructed.
- There are several early listings of chemical substances, including Valentinus' Alchemy Table and Lavoisier's Table of Simple Substances (1789). In 1803 Dalton proposed that matter consists of discrete atoms that combine in fixed ratios, stoichiometry, to form chemical elements. Thus, Dalton's list of chemical elements, plus mass data, must be included in any top ten listing:
- If you examine the periodic tables from Antiquity to 1899, you will see that from about 1830 onwards, proto-periodic tables were coming thick and fast. Significant developments include: Daubeny's Teaching Display Board of Atomic Weights (1831), Chancourtois Telluric Helix (1862) and Newlands octaves (1864).
But, it was Mendeleev's Tabelle I that was first near complete periodic table formulation of the then known elements (no Group 18 rare gasses, note). Crucially, Mendeleev identified gaps and was able to make predictions about the chemical properties of the missing substances. Plus, Mendeleev promoted his ideas with great energy:
- Werner's 1905 Periodic Table is remarkably modern looking. The formulation is a long form that separates transition metals and rare earths, but he guessed wrong on how many existed:
- Janet's Left Step formulation of 1928 is one for the purists as it clearly shows the chemical elements arranged into s, p, d & f-blocks of the recently developed quantum mechanical description of atomic structure:
- The modern (and commonly employed) periodic table is obtained by transforming Janet's Left Step into the modern long form periodic table by rearranging the blocks around. This transformational mapping is discussed in some detail here.
The long form and medium form PTs have electronegativity trending from top-right (electronegative) to bottom left (electropositive), and many aspects of periodicity corollate with electronegativity: atomic radius, first ionisation energy, etc.
Thus, the long form and medium form periodic tables are commonly used in the classroom:
An Alternative Formulation
The internet database contains many, many alternative formulations, and these are often spiral and/or three dimensional. These exemplified by the 1965 Alexander DeskTopper Arrangement. To see the variety of formulations available, check out the Spiral & Helical and 3-Dimensional formulations in the database:
Non-Chemistry PTs
The periodic table as a motif is a useful and commonly used infographic template for arranging many types of object with, from 50 to 150 members.
There are numerous examples in the Non-Chemistry section where dozens of completely random representations can be found:
- Adobe Illustrator Shortcuts
- Adult Positions
- Airline Customer Reviews
- Beer Styles
- And, chosen more or less at random, European Nations:
Year: 2013 | PT id = 619 |
Averaged Ionisation Potential Periodic Table
By Leland Allen, a representation of the periodic table with the third dimension of energy derived from the averaged ionisation potentials of the s and p electrons. (Allen suggested that this was a direct measure of electronegativity). From J. Am. Chem. Soc. 1989, 111, 9004:
Year: 2018 | PT id = 774 |
First Ionisation Energy to the Standard Form Periodic Table
There is debate amongst the cognoscenti about the 'best' representation of the periodic table, and how this 'best' formulation can be explained by [rationalized by] quantum mechanics (QM).
Many feel that the Janet PT formulation, the 'Left Step', is the ideal QM PT, but this formulation does not show periodicity very well, and there are issues with the placement of H, He, Be which spill over into questions about their placement in the standard form PT (the periodic table used in classrooms and textbooks around the world).
However, it is possible to get to the conventional standard form PT directly from the first ionisation energy data, where the 1st ionisation energy is the energy required to convert a gas phase atom (M) into its gas phase positive ion plus electron.
M(g) → M+(g) + e–
The process involves:
- taking the 1st ionisation data plot for the elements H to Xe (Z = 1 to 36)
- rotate 90° clockwise and stretch
- move the atoms horizontally into columns
Note that a similar logic can be applied to atomic radius and electronegativity data.
However, there are issues about the measurement of atomic radius, because atoms are 'soft at their edges', and gas phase atomic radius is not precisely defined. And, electronegativity is a derived parameter.
By Mark Leach
Year: 2018 | PT id = 929 |
Acid-Base Behavior of 100 Element Oxides
Acid-Base Behavior of 100 Element Oxides: Visual and Mathematical Representations by Mikhail Kurushkin and Dmitry Kurushkin. J. Chem. Educ. 95, 4, 678-681.
A novel educational chart that represents the acid-base behavior of 100 s-, p-, d-, and f-element oxides depending on the element's electronegativity and oxidation state was designed. An updated periodic table of said oxides was developed. A mathematical criterion based on the chart was derived which allows prediction of the behavior of unfamiliar oxides:
Year: 2020 | PT id = 1117 |
Correlation of Electron Affinity (F) with Elemental Orbital Radii (rorb)
From Jour. Fac. Sci., Hokkaido Univ., Ser. IV. vol. 22, no. 2, Aug., 1987, pp. 357-385, The Connection Between the Properties of Elements and Compounds; Mineralogical-Crystallochemical Classification of Elements by Alexander A. Godovikov & Yu Hariya and expanded by René Vernon who writes.
René Vernon writes:
I was delighted to read about two properties that account for nearly everything seen in the periodic table.
Two properties
While researching double periodicity, I happened upon an obscure article, which simply correlates electron affinity with orbital radius, and in so doing reproduces the broad contours of the periodic table. Having never thought much about the value or significance of EA, and its absence of easily discernible trends, I was suitably astonished. The authors left out the Ln and An and stopped at Bi. They were sitting on a gold mine but provided no further analysis.Development
I added the data up to Lr, updated the EA values, and have redrawn their graph. It is a thing of beauty and wonderment in its simplest sufficient complexity and its return on investment. I've appended 39 observations, covering all 103 elements.
Observations
- Very good correspondence with natural categories
- Largely linear trends seen along main groups; two switchbacks seen in group 13; also falloffs (6p sub-shell) seen in groups 14-17
- First row anomalies seen for Li (in amphoteric territory), Be (ditto), C (misaligned), N (in noble gas territory), O (misaligned), F (ditto) and He (ditto)
- For group 13, the whole group is anomalous, no doubt due to the scandide contraction impacting Ga and the double whammy of the lanthanide and 5d contraction impacting Tl
- Nitrogen was called a noble gas before the discovery of the real noble gases and appropriately enough falls into that territory
- Rn is metallic enough to show cationic behaviour and falls just outside of noble gas territory
- F and O are the most corrosive of the corrosive nonmetals
- The rest of the corrosive nonmetals (Cl, Br and I) are nicely distributed, across the border from F
- The rest of the simple and complex anions, funnily enough, comprise the intermediate nonmetals
- The metalloids are nicely aligned; Ge falls a little outside of the metalloid line, being still occasionally referred to as a metal; Sb, being the most metallic of the metalloids falls outside the border; At is inside; Po is just outside
- Pd is located among the nonmetals due to its absence of 5s electrons; see here
- The proximity of H to Pd is astonishing given the latter's capacity to adsorb the former
- The post-transition metals (PTM) form an "archipelago of amphoterism" bounded by transition metals: Ni and C to the west; Fe and Re to the south; V, Tc and W to the east; noble metals to the north
- Curiously, Zn, Cd, and Hg are collocated with Be, and distant from the PTM and the TM proper (aside from Mn)
- Zn is shown as amphoteric, which it is. Cd is shown as cationic but is not too far away from amphoteric territory; it does show amphoterism, reluctantly; Hg is shown as amphoteric which is the case, weakly, for HgO, as is the congener sulfide HgS, which forms anionic thiomercurates (such as Na2HgS2 and BaHgS3) in strongly basic solutions
- The ostensibly noble metals are nicely delineated; Ag is anomalous given its greater reactivity; Cu, as a coinage metal, is a little further away
- The proximity of Au and Pt to the halogen line is remarkable given the former's capacity to form monovalent anions
- The ferromagnetic metals (Fe-Co-Ni) form a nice line
- The TM from groups 4-12 form switchback patterns e.g. Ti-Zr and the switchback to Hf
- The refractory metals, Nb, Ta, Mo, W and Re are in a wedge formation
- Tc is the central element of the periodic table in terms of mean radius and EA values; V is close, Cr is a little further away
- Ti is just inside the basic cation line; while Ti(IV) is amphoteric, Ti3+ is ionic
- Sc-Y-La shows a main group pattern up to La, when there is a switchback to Ac
- Sc-Y-Lu-Lr shows a TM switch back pattern
- La, and to lesser extent Ce are rather separated from the rest of the Ln, consistent with Restrepo and here.
- Sc and Lu are close to the amphoteric territory and are both in fact, weakly amphoteric
- The post-cerium Ln and An (but for Th) all fall within basic cation territory
- EA values for the An are estimates and need to be treated with due caution
- The light actinides (Th to Cm) occupy a tight locus, with the exception of Th, where the 5f collapse is thought to occur, and Pu, which sits on the border of 5f delocalisation and localisation
- While the light actinides U to Cm are shown as being cationic they are all known in amphoteric forms
- The heavy actinides, Bk to Lr, are widely dispersed
- All the Ln, bar Tm, are located within close proximity of the light An locus; Tm is the least abundant stable Ln
- The gap between La and Ce, and rest of the Ln is consistent with Restrepo's findings and here
- Nobelium in this edition of the chart falls off the bottom, having a radius 1.58 (cf Es) and an EA of -2.33
- There is an extraordinary alignment between He and the Group 2 metals
- Magnesium is on the cationic-amphoteric boundary; some of its compounds show appreciable covalent character
- Li, being the least basic of the alkali metals, is located just outside the alkalic zone; Li compounds are known for their covalent properties
- The reversal of the positions of Fr and Cs is consistent with Cs being the most electronegative metal
- A similar, weaker pattern is seen with Ba and Ra.
Conclusion
So there it is, just two properties account for nearly everything.
Click images below to enlarge:


Year: 2020 | PT id = 1122 |
Vernon's Constellation of Electronegativity
René Vernon has created a "Constellation of Electronegativity" by plotting Electronegativity against Elemental Orbital Radii (rorb)
Observations on the EN plot:
- The results are similar to the orbital radii x EA plot, although not quite as clear, including being more crowded
- Very good correspondence with natural categories
- Largely linear trends seen along groups 1-2, 17 and 15-18 (Ne-Rn)
- First row anomaly seen for He (or maybe not since it lines up with the rest of group 2)
- For group 13, the whole group is anomalous
- For group 14 , the whole group is anomalous no doubt due to the scandide contraction impacting Ge and the double whammy of the lanthanide and 5d contraction impacting Pb
- F and O are the most corrosive of the corrosive nonmetals
- The rest of the corrosive nonmetals (Cl, Br and I) are nicely aligned with F
- The intermediate nonmetals (IM) occupy a trapezium
- Iodine almost falls into the IM trapezium
- The metalloids occupy a diamond, along with Hg; Po is just inside; At a little outside
- Rn is metallic enough to show cationic behaviour and falls into the metalloid diamond
- Pd is located among the nonmetals
- The proximity of H to Pd is again (coincidentally?) curious given the latter's capacity to adsorb the former
- The post-transition metals occupy a narrow strip overlapping the base of the refractory metal parallelogram
- Curiously, Zn, Cd, and Hg (a bit stand-off-ish) are collocated with Be, and relatively distant from the PTM and the TM proper
- The ostensibly noble metals occupy an oval; curiously, W is found here; Ag is anomalous given its greater reactivity; Cu, as a coinage metal, is a little further away
- Au and Pt are nearest to the halogen line
- The ferromagnetic metals (Fe-Co-Ni) are colocated
- The refractory metals, Nb, Ta, Mo, W and Re are in a parallelogram, along with Cr and V; Tc is included here too
- Indium is the central element of the periodic table in terms of mean orbital radius and EN; Tc is next as per the EA chart
- The reversal of He compared to the rest of the NG reflects #24
- All of the Ln and An fall into an oval of basicity, bar Lr
- The reversal of the positions of Fr and Cs is consistent with Cs being the most electronegative metal
- A similar, weaker pattern is seen with Ba and Ra.

Year: 2020 | PT id = 1165 |
Vernon's (Partially Disordered) 15 Column Periodic Table
A formulation by René Vernon, who writes:
"Here is a 15-column table which is a hybrid of a Mendeleev 8-column table and an 18-column standard table. The key relocations are the p-block nonmetals to the far left; and the coinage and post-transition metals under their s and early d-block counterparts.
"Taking a leaf out of Mendeleev's playbook, I ignored atomic number order when this seemed appropriate. It's refreshing to see the traditional horizontal gaps between blocks disappear. (DIM did not like these.)
"Since Dias (2004, see references below) reckoned a periodic table is a partially ordered set forming a two-dimensional array, I believe I now have a partially ordered table that is partially disordered twice over.
"The table has some curious relationships. Equally, some relationships seen in the standard form are absent. The Group 2, 3, and aluminium dilemmas disappear. This confirms my impression that such dilemmas have no intrinsic meaning. Rather, their appearance or non-appearance is context dependent."
Notes & references below.
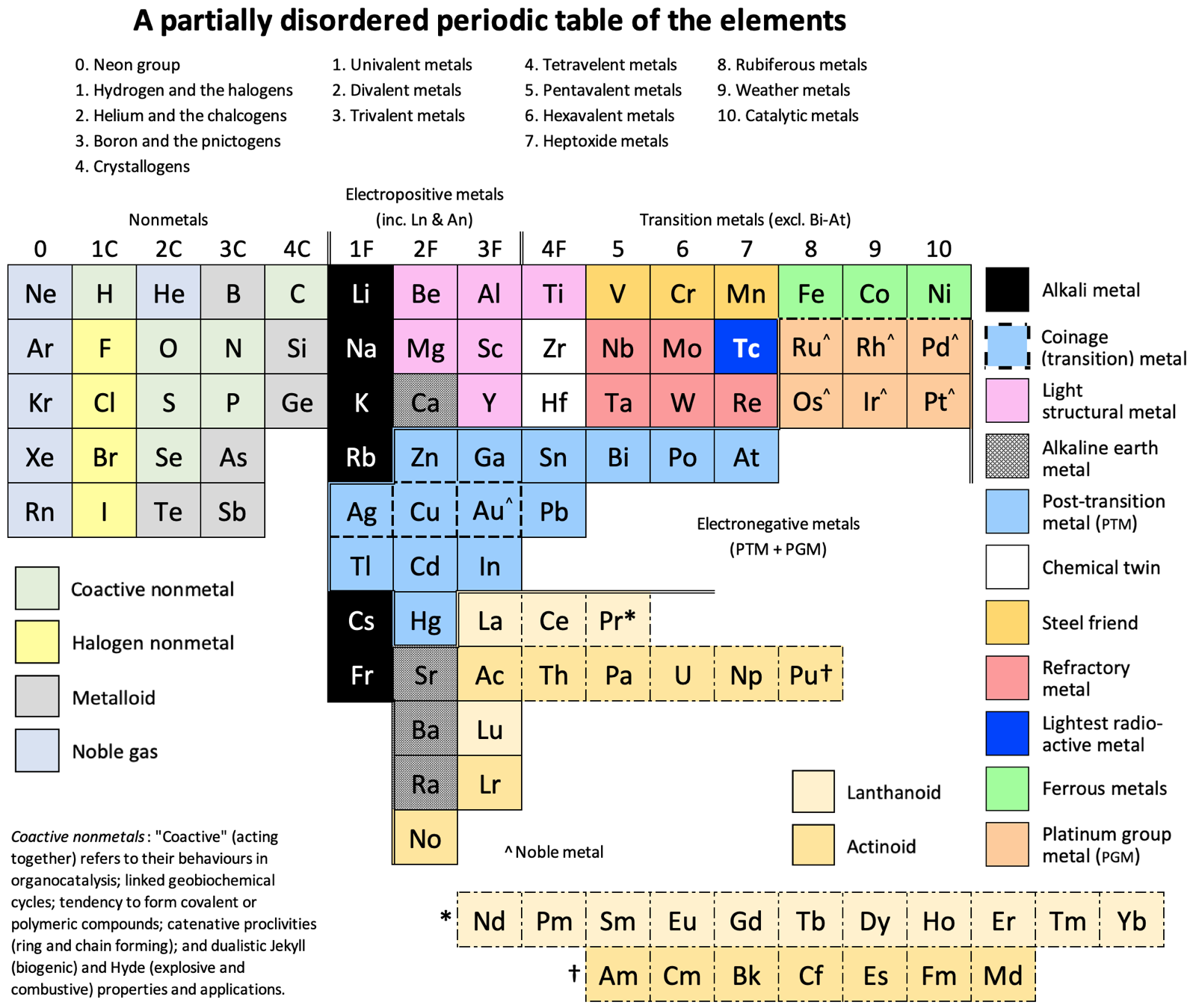
Groups 1 to 4 have either a C or F suffix where C (nonmetal) is after the importance of carbon to our existence; and F (metal) is for the importance of iron to civilisation.
Groups 1C and 1F present the greatest contrast in nonmetallic and metallic behaviour.
Coactive Nonmetals: They are capable of forming septenary heterogeneous compounds such as C20H26N4O10PSSe.
Group 2C: Helium is shaded as a noble gas. "Heliox" is a breathing gas mixture of helium and oxygen used in saturation diving, and as a medical treatment for patients with difficulty breathing.
Group 3C: Boron over nitrogen looks odd. Yet one boron atom and one nitrogen atom have the same number of electrons between them as two adjacent carbon atoms. The combination of nitrogen and boron has some unusual features that are hard to match in any other pair of elements (Niedenzu & Dawson 1965).
Boron and phosphorus form a range of ring and cage compounds having novel structural and electronic properties (Paine et al. 2005).
Metalloids. I treat them here as nonmetals given their chemistry is predominately that of chemically weak nonmetals.
Metals: The labels electropositive; transition; and electronegative are adapted from Kornilov (2008).
Group 1F: Monovalent thallium salts resemble those of silver and the alkali metals.
An alloy of cesium (73.71%), potassium (22.14%) and sodium (4.14%) has a melting point of –78.2°C (–108.76°F) (Oshe 1985).
Silver, copper, and gold, as well as being the coinage metals, are borderline post-transition metals.
Group 2F: Beryllium and magnesium are not in fact alkaline earths. Beryllium is amphoteric rather than alkaline; magnesium was isolated in impure form from its oxides, unlike the true alkaline earths. The old ambiguity over whether beryllium and magnesium should go over calcium or zinc has gone.
Nobelium is here since +2 is its preferred oxidation state, unlike other actinoids.
Group 3F: Aluminium is here in light of its similarity to scandium (Habishi 2010).
InGaAsP is a semiconducting alloy of gallium arsenide and indium phosphide, used in lasers and photonics.
There is no Group 3 "issue" since lanthanum, actinium, lutetium and lawrencium are in the same family.
Gold and aluminium form an interesting set of intermetallic compounds known as Au5Al2 (white plague) and AuAl2 (purple plague). Blue gold is an alloy of gold and either gallium or indium.
Lanthanoids: The oxidation state analogies with the transition metals stop after praseodymium. That is why the rest of lanthanoids are footnoted in dash-dot boxes.
Actinoids: The resemblance to their transition metal analogues falters after uranium, and peters out after plutonium.
Group 4F: It's funny to see titanium—the lightweight super-metal—in the same group as lead, the traditional "heavy" metal.
This is the first group impacted by the lanthanoid contraction (cerium through lutetium) which results in the atomic radius of hafnium being almost the same as that of zirconium. Hence "the twins".
The chemistry of titanium is significantly different from that of zirconium and hafnium (Fernelius 1982).
Lead zirconate titanate Pb[ZrxTi1–x]O3 (0 ≤ x ≤ 1) is one of the most commonly used piezo ceramics.
Group 5: Bismuth vanadate BiVO4 is a bright yellow solid widely used as a visible light photo-catalyst and dye.
Steel Friends: The name is reference to their use in steel alloys. They have isoelectronic soluble oxidizing tetroxoanions, plus a stable +3 oxidation state. (Rayner-Canham 2020).
Ferromagnetic Metals: The horizontal similarities among this triad of elements (as is the case among the PGM hexad) are greater than anywhere in the periodic table except among the lanthanides (Lee 1996). The +2 aqueous ion is a major component of their simple chemistry (Rayner-Canham 2020).
Group 8: "Rubiferous metals" (classical Latin rubēre to be red; -fer producing) is from the reddish-brown colour of rust; the most prevalent ruthenium precursor being ruthenium trichloride, a red solid that is poorly defined chemically but versatile synthetically; and the red osmates [OsO4(OH)4]?2 formed upon reaction by osmium tetroxide with a base.
Group 9: "Weather metals" comes from the use of cobalt chloride as a humidity indicator in weather instruments; rhodium plating used to "protect other more vulnerable metals against weather exposure as well as against concentrated acids or acids fumes" (Küpfer 1954); and the "rainbow" etymology of iridium.
Group 10: "Catalytic metals" is after a passage in Greenwood and Earnshaw, "They are... readily obtained in finely divided forms which are catalytically very active." (2002). Of course, many transition metals have catalytic properties. That said, if you asked me about transition metal catalysts, palladium and platinum would be the first to come to mind. Group 10 appear to be particularly catalytic.
References:
- Dias JR 2004, "The periodic table set as a unifying concept in going from benzenoid hydrocarbons to fullerene carbons", in DH Rouvray & RB King (eds.), The periodic table: into the 21st century, Institute of Physics Publishing, Philadelphia, pp. 371–396 (375)
- Fernelius WC 1982, "Hafnium," J. Chem. Educ. vol. 59, no. 3, p. 242
- Greenwood NN & Earnshaw A 2002, Chemistry of the elements, 2nd ed., Butterworth-Heinemann, Oxford, p. 1148
- Habashi F 2010, "Metals: typical and less typical, transition and inner transition", Foundations of Chemistry, vol. 12, pp. 31–39
- Lee JD 1996, Concise inorganic chemistry, 5th ed., Blackwell Science, Oxford, p. 753
- Kornilov II 1965, "Recent developments in metal chemistry", Russian Chemical Reviews, vol. 34, no. 1, p. 33
- Küpfer YJ 1954, "Rhodium uses in plating", Microtecnic, Agifa S.A., p. 294 Niedenzu K & Dawson JW 1965, Boron-nitrogen compounds, Springer, Berlin, preface
- Oshe RW (ed.) 1985, "Handbook of thermodynamic and transport properties of alkali metals", Blackwell Scientific, Oxford, p. 987
- Paine et al. 2005, "Recent developments in boron-phosphorus ring and cage chemistry", in Modern aspects of main group chemistry, M Lattman et al. (eds.), ACS Symposium Series, American Chemical Society, Washington DC, p. 163
- Rayner-Canham G 2020, The periodic table: Past, present, and future, World Scientific, Singapore
Year: 2021 | PT id = 1183 |
Crustal Abundance vs. Electronegativity
A chart by René Vernon of Elemental Abundance (g/kg log10) vs. Electronegativity, H to Bi.
René writes:
Below is a remarkable XY chart where x = electronegativity and y = crustal abundance (log10). It stops at the end of the s-process, at Bi. The abundance figures are from the CRC Hanbook of Physics and Chemistry (2016-2017).
I say remarkable as I had little idea what the chart would end up looking like when I started plotting the values.
As well as its coloured regions, I've marked out track lines for six of the main groups and one for group 3.
Observations
The rose-coloured arc on the left encompasses the pre-transition metals i.e. the alkali and alkaline earth metals and aluminium, followed by, in the orange rectangle, the rare earth metals. Opposite these regions, along the southern boundary of the green paddock, are the halogens.
In the pale yellow field sheltered by the pre-transition metals and the REM, are the 3d transition metals and, in the white corral, are 4d and 5d base transition metals. Opposite these regions, in the green paddock, are the core nonmetals H, C, N, O, P and S, with Se as an outlier.
Following in the grey blob are the post-transtion or poor metals, immediately adjacent to the bulk of the metalloids or poor nonmetals.
Finally, in the light blue patch, the noble metals are complemented by the noble gases frolicking in the open.
Abundance tends to decrease with increasing Z. Notable exceptions are Li, B, N and Si.
Curiosities
- H and P are almost on top of one another
- The proximity of Be to the post-transition metals, and its relative scarcity in the crust
- The metalloids, with their intermediate values of electronegativity, go down the middle. At the same time they span nearly the full range of abundance.
- B-Ga-Sc-Y-La are in a row
- N falls along the halogen line
- The abundance of O and Si, which we see in the form of silica
- F is more abundant in the crust than 85 percent of metals
- Al is the most abundant metal. Al and Fe are in the same vicinity: "Curiously, the chemistry of aluminium also resembles that of the iron(III) ion... These similarities may be ascribed to the same 3+ charge and near-identical ion radii (and hence charge density)." (Rayner-Canham 2020, p. 191)
- The abundance of Ar compared to the rest of the noble gases. Apparently this is influenced by the radioactive decay of potassium-40 in Earth's core, which is considered one of the main sources of heat powering the geodynamo that generates Earth's magnetic field. It has been suggested that a large amount of Ar may be present in the core, as the compound ArNi with an L11 Laves structure (similar to an intermetallic phase, and related to a cubic close packed lattice). ArNi is stabilised by notable electron transfer from Ni to Ar, changing their electron configurations toward 3d7 and 4s1. (Adeleke et al. 2019)
- Ti, a light yet strong metal, is about 2,500 times as abundant as Sn, a weak heavy metal
- Zn is an outlaw post-transition metal
- The most active 4d-5d transition metals (Zr, Hf) occupy a boundary overlap with the rare earth metals
- Ag, which has a largely main-group chemistry, is located in the PTM region. It is about 20 times as abundant as the noble meals
- Re is an outlaw noble metal
Comment
I was intrigued by the article referring to Ni and Ar, and the suggestion of Ar becoming somewhat anionic, albeit in extreme conditions (140 GPa, 1500 K)
References
- Adeleke AA, Kunz M, Greenberg E, Prakapenka VB, Yao Y, Stavrou R 2019, A high-pressure compound of argon and nickel: Noble gas in the Earth's core?, ACS Earth and Space Chemistry, vol. 3 no. 11, pp. 2517-2544, https://pubs.acs.org/doi/10.1021/acsearthspacechem.9b00212
- Rayner-Canham G, 2020, The periodic table: Past, present, future, World Scientific, Singapore
Correlations
I wasn't looking for these but they at least exist as follows:
- Metals with lower EN, i.e. < 1.7, or active nonmetals with higher EN, tend to be concentrated in silicate or oxide phases that are more easily found in the crust due to their lower density, and hence have higher abundances.
- Metals with moderate EN 1.7 to 2.1, say the later transition metals and post-transition metals, tend to form sulfide liquid phases; are less easily found in the crust due to their relatively higher densities; and are less abundant by about two orders of magnitude compared to the metals found in silicate or oxide phases.
- Metals with EN > 2.2, i.e. the noble metals, have an affinity for a metallic liquid phase, and are depleted in the crust since they generally sank to the core and hence have very low abundances. They are about two orders of magnitude less abundant than the sulfide metals.
My references are:
- Cox PA 1997, The elements: Their origin, abundance and distribution;
- Gill R 2014, Chemical fundamentals of geology and environmental geoscience;
- White WA 2020, Geochemistry
Thus the abundance of the metals in the crust tends to fall with increasing EN.
- For the nonmetals, the relative average abundance proportions are about 5: 700: 250: 1 for, respectively, the metalloids; the core nonmetals H, C, N, P, S, and Se; the halogen nonmetals; and the noble gases. Si and O were left out as outliers, in terms of their massive abundances.
- Thus, metalloids aside, the abundance of the nonmetals tends to fall with increasing EN. I don't know what's going on with the metalloids.
- The chart may prompt some further appreciative enquiry:
- In the case of exceptions to the initial three generalisations why do these occur?
- Why is Li so rare, compared to the other alkali metals?
- Why is Si good at forming a planetary crust?
An answer from L. Bruce Railsback, creator of the Earth Scientist's Periodic Table https://www.meta-synthesis.com/webbook/35_pt/pt_database.php?PT_id=142:
"I think I can answer one of the questions. 'Why is Si good at forming a planetary crust?' – because it's so bad at staying in the core. Silicon isn't sufficiently metallic to stay in the core. Even in the mantle and crust, it doesn't go into non-metal solids well: in cooling magmas, it's only a lesser member of the early-forming minerals (e.g., Mg2SiO4, forsterite, where it's outnumbered two to one). The mineral only of Si as a cation, SiO2 (quartz), is the LAST mineral to form as a magma cools, in essence the residuum of mineral-forming processes. At least some this thinking is at Bowen's Reaction Series and Igneous Rocks at http://railsback.org/FundamentalsIndex.html#Bowen"
- Why do the metalloids span such a wide range of abundances?
- If H is supposed to make up ca. 74% of the universe why does it have the same abundance in the Earth's crust as P?
- In what form is H found in Earth's crust—water, hydroxides?
- If H is supposed to make up ~ 74% of the universe why does it have the same abundance in the Earth's crust as P?
- Are there any chemical similarities between H and P, given both have some metalloidal character? The have virtually identically electron affinities. H is sometimes positioned above B due to chemical similarities. It then forms a diagonal relationship with C, which in turn has a diagonal relationship with P, which has a diagonal relationship with Se e.g. P reacts with Se to form a large number of compounds characterised by structural analogies derived from the white phosphorus P4 tetrahedron.
- The rare earth metals are relatively rare, having an average abundance of 1% that of the 3d metals. That being so, why is their rareness sometimes questioned? Why does the crustal abundance of the REM plummet by two orders of magnitude towards the end of the lanthanides?
Which Electronegativity Scale?
The wide variety of methods for deriving electronegativities tend to give results similar to one another.
Year: 2021 | PT id = 1190 |
Electronegativity: A Three-Part Wave
René Vernon points out that although there is a general trend in increasing electnegativity from Cs to F, there is actually an s-curve in the data.
Electronegativity across groups 1 to 18 appears to a show a three-part wave-like pattern.
There is a rise from group 1 to group 6, followed by a fall at group 7. I guess for group 7 that the EN for Mn is based on +2 and in this state Mn has five 3d electrons. The EN for Tc and Re are presumably based on +7, in which they notionally have underlying [Kr] and [Xe] cores.
There is rise from 7 to 8 (why?); a mesa from 8 to 11 (why?) that includes the PGM; and a fall at group 12. The fall may be influenced by group 12 having a full d shell; ditto group 13.
There is a rise from 13 to 18. Whereas in group 13 there is ionic chemistry in the form of the cations of Al to Tl this is not the case for C, Si, and Ge in group 14. Sn is reluctant to form a cation expect at pH < 1, and there is no Pb4+ cation.
The R2 value of 0.9739 is a best fit value for a second order polynomial. R2 for a straight line is 0.786

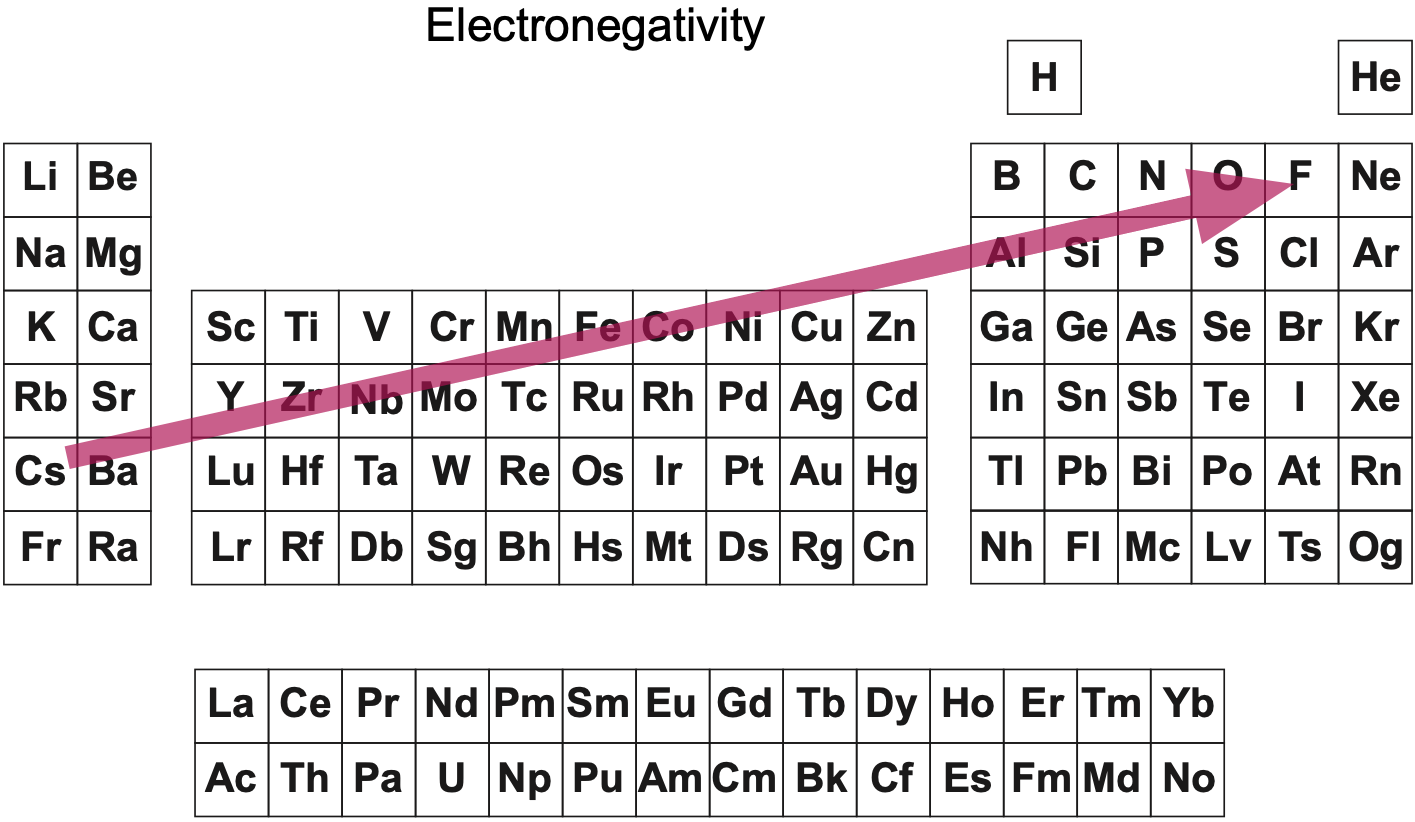
Year: 2022 | PT id = 1241 |
Electronegativity Seamlessly Mapped Onto Various Formulations of The Periodic Table
A discussion on the Google Groups Periodic Table Discussion List, involving a René Vernon, Nawa Nagayasu & Julio Samanez (all contributors this database) lead to the development of the representations below, showing electronegativity seamlessly mapped onto a modified Left-Step Periodic Table:
Nawa Nagayasu has mapped electronegativity to Mendeleeve's formulation:
Nawa Nagayasu has mapped electronegativity onto other formulations, Julio's Binode Spiral:
and the "conventional", short, medium and long forms of the periodic table with hydrogen above and between B & C which show the botom-right-to-top-left electronegativity trend:
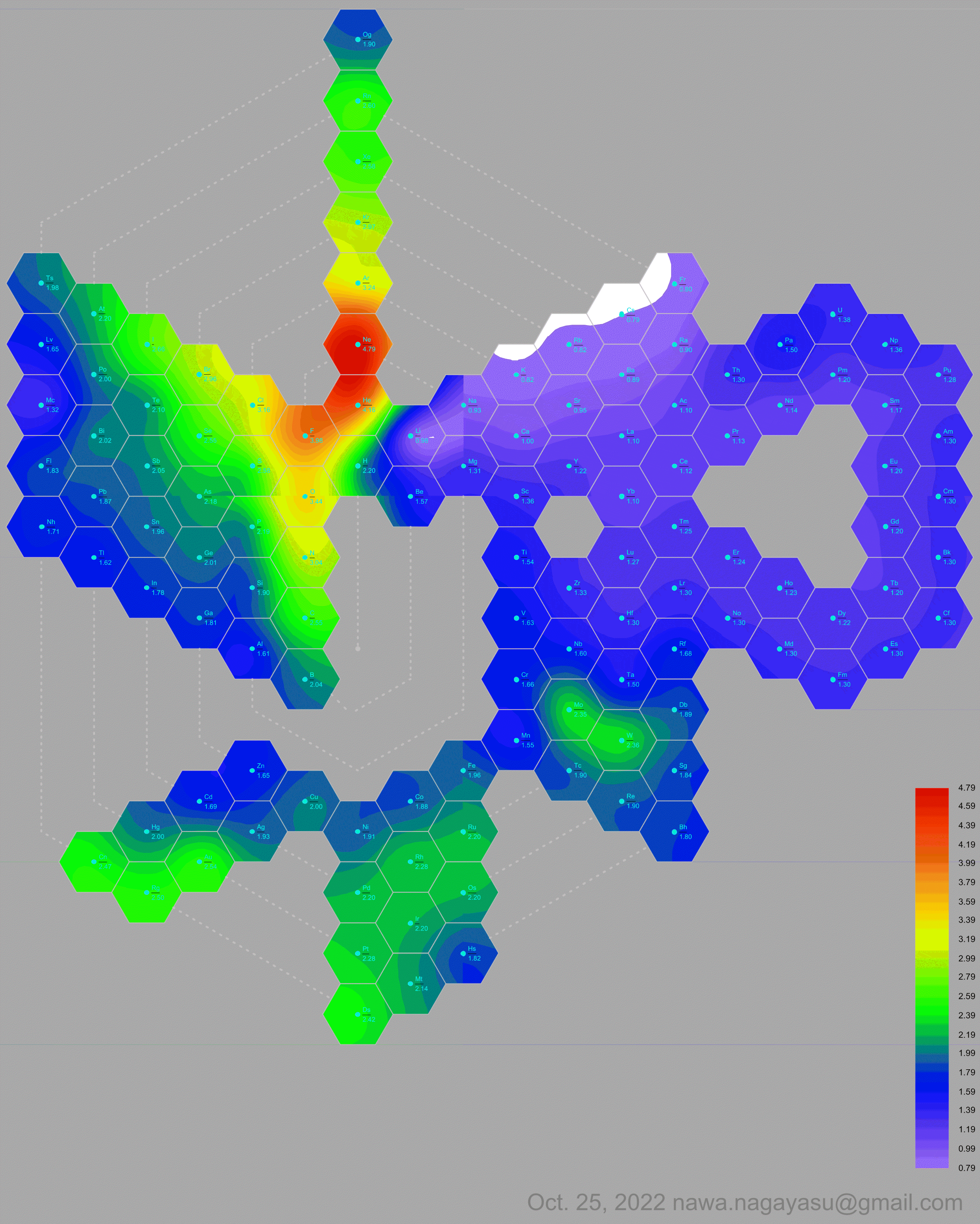
René Vernon's 777 Periodic Wedding Cake:
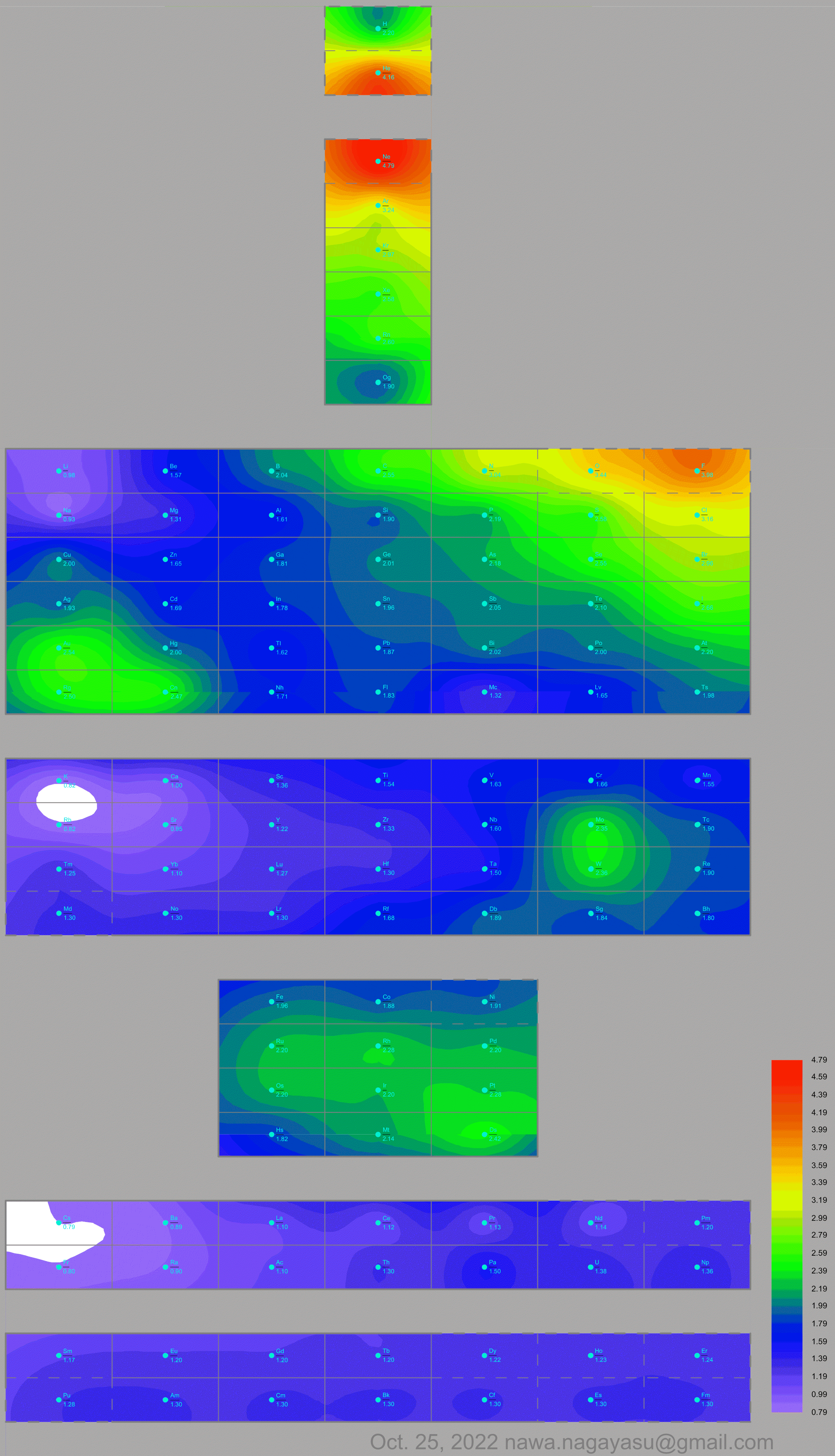
Valery Tsimmerman's ADOMAH formulation:

Valery Tsimmerman's ADOMAH tetrahedron (in a glass cube) formulation:
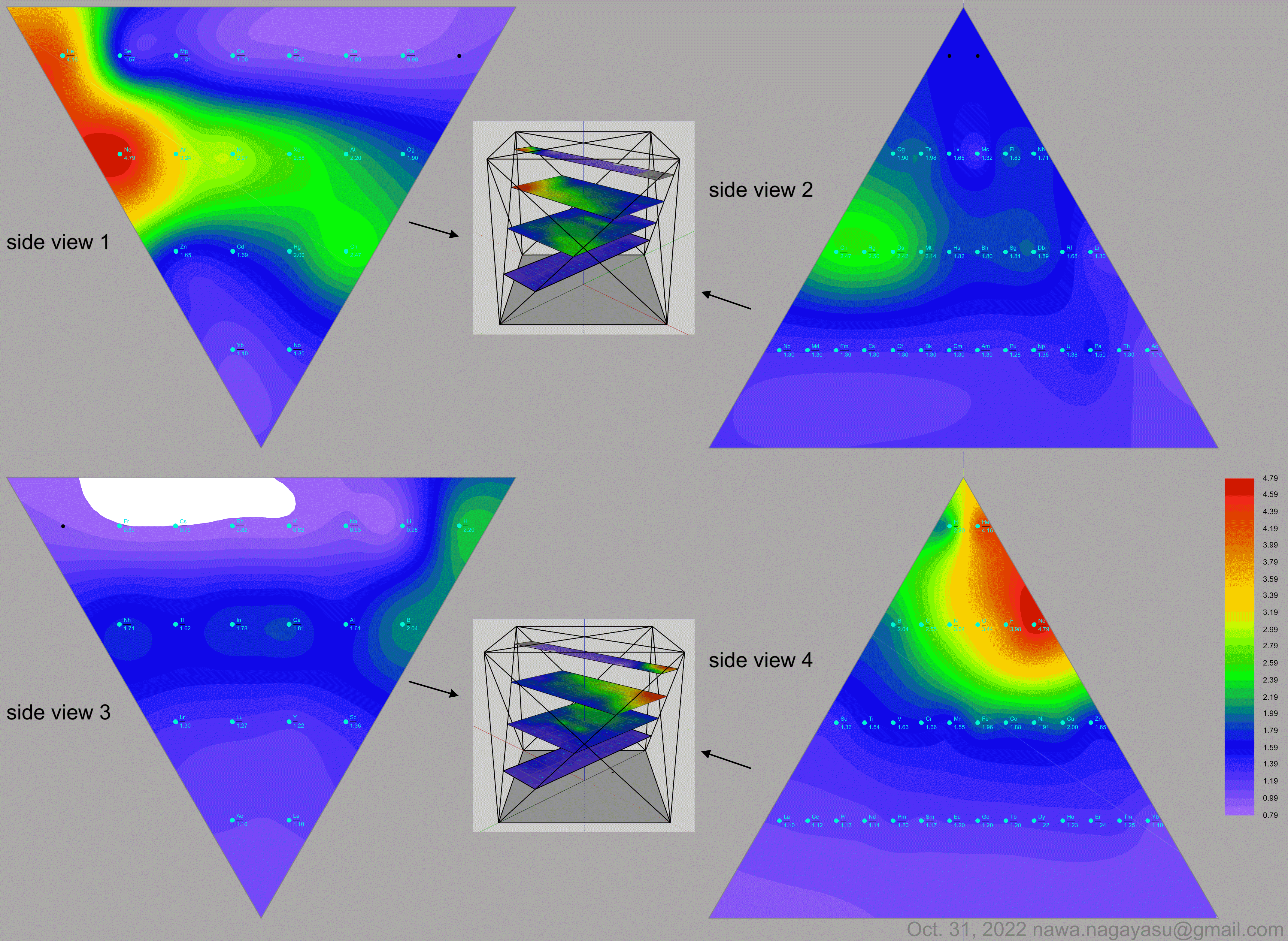
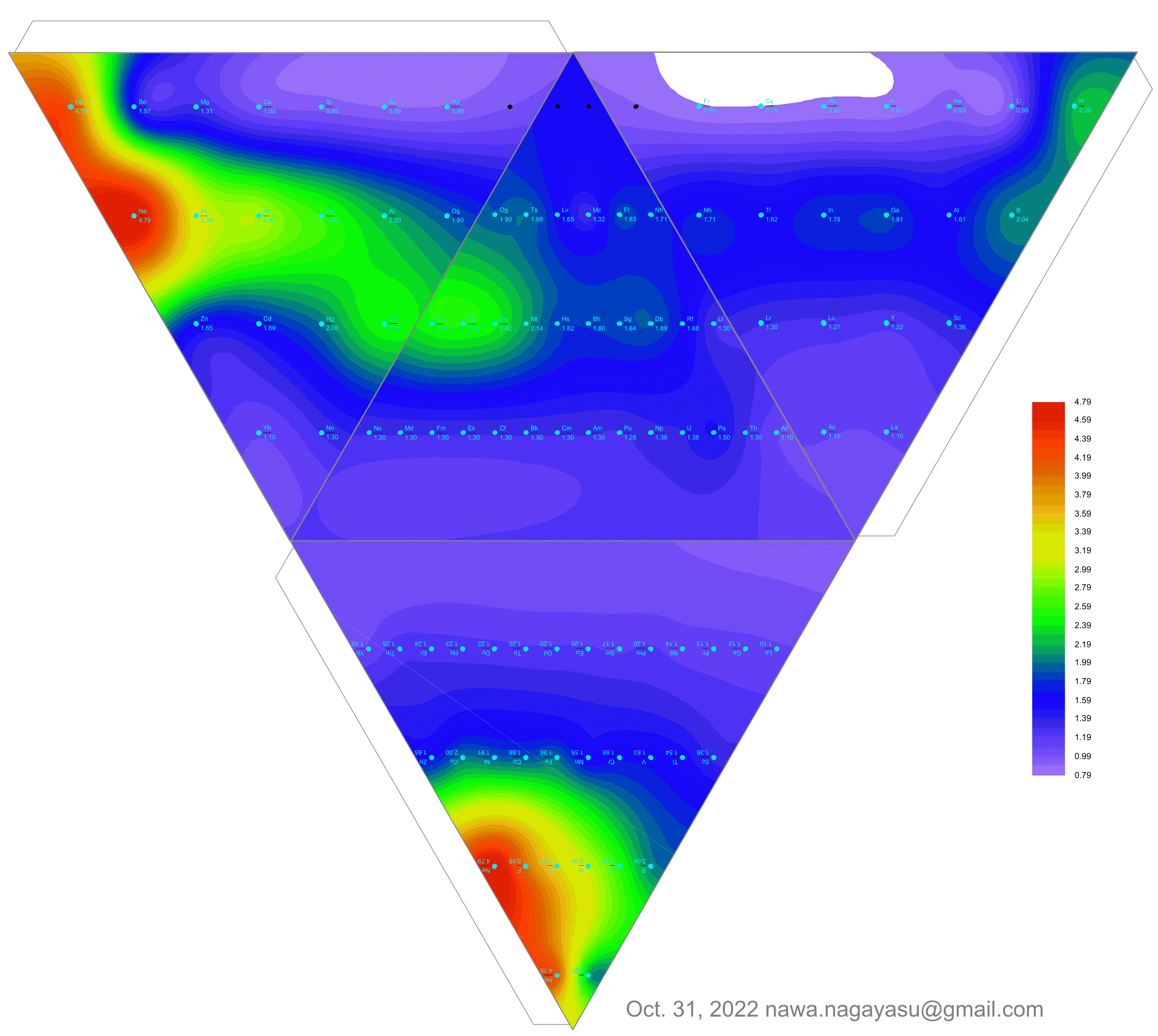

Year: 2022 | PT id = 1254 |
99 Elements Sorted by Density & Electronegativity
René Vernon writes:
"A little while I ago I noticed that a scatter plot of EN (revised Pauling) and density values of the elements resulted in a nice distribution, as per the table below.
"According to Hein and Arena (2013) nonmetals have low densities and relatively high EN values; the table bears this out. Nonmetallic elements occupy the top left quadrant, where densities are low and EN values are relatively high. The other three quadrants are occupied by metals. Of course, some authors further divide the elements into metals, metalloids, and nonmetals although Odberg argues that anything not a metal is, on categorisation grounds, a nonmetal.
Note 25 says:
(a) Weighable amounts of the extremely radioactive elements At (element 85), Fr (87), and elements with an atomic number higher than Es (99), have not been prepared.
(b) The density values used for At and Fr are theoretical estimates.
(c) Bjerrum (1936) classified "heavy metals" as those metals with densities above 7 g/cm^3.
(d) Vernon (2013) specified a minimum electronegativity of 1.9 for the metalloids.
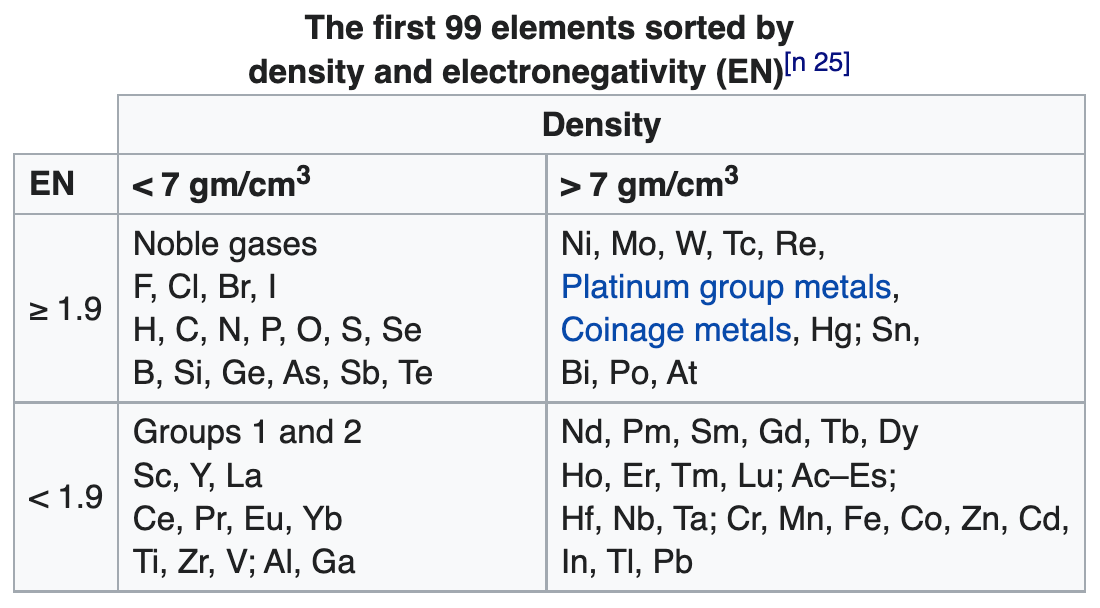
- Bjerrum, N (1936). Bjerrum’s Inorganic Chemistry. London: Heinemann
- Hein, M; Arena, S (2013). Foundations of College Chemistry. Hoboken: John Wiley & Sons. pp. 226, G-6. ISBN 978-1-118-29823-7.
- Oderberg DS 2007, Real Essentialism, Routledge, New York, ISBN 978-1-134-34885-5
- Vernon R 2013, "Which elements are metalloids?", Journal of Chemical Education, vol. 90, no. 12, 1703?1707, doi:10.1021/ed3008457
![]() |
![]() |
![]() |
What is the Periodic Table Showing? | Periodicity |
© Mark R. Leach Ph.D. 1999 –
Queries, Suggestions, Bugs, Errors, Typos...
If you have any:
Queries
Comments
Suggestions
Suggestions for links
Bug, typo or grammatical error reports about this page,please contact Mark R. Leach, the author, using mark@meta-synthesis.com
This free, open access web book is an ongoing project and your input is appreciated.